Breaking Down the Multiple Hazards of Volcanic Eruptions
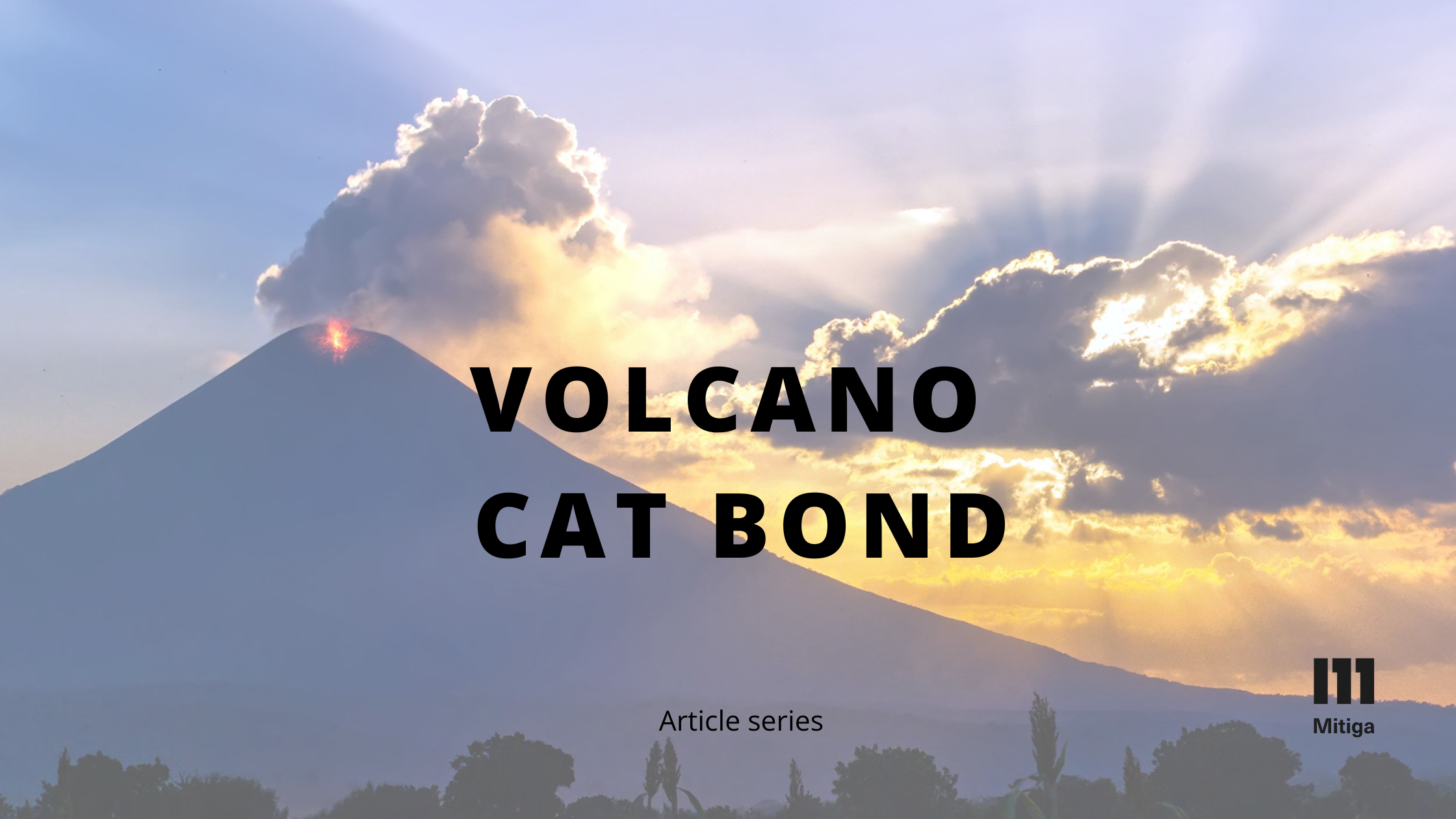
Volcanoes act as gateways in the Earth’s crust through which molten rock can rise from depth to the surface. Molten rock is referred to as magma and often exceeds temperatures of 1000°C - when it exits the crater, we call it lava. Once free from the constraints of the magma chamber and conduit, lava can spread in various ways. It may flow slowly but unstoppably in lava flows, or it may erupt in a massive explosion that fragments the lava and propels it high into the atmosphere. The mechanisms associated with volcanic eruptions are complex, multifaceted, and not yet fully understood – and they also pose significant dangers to human life.
While volcanic eruptions are often listed as a single natural hazard, each volcano and eruption encompass multiple hazardous phenomena that act on various temporal and spatial scales and have different potentials for damage. These volcanic hazards can interact with and give rise to one another, occurring simultaneously or successively.
In this article, we will provide a brief overview of the multiple and unique hazards associated with volcanic eruptions.
Ballistic Bombs
Nearly every eruption ejects fragments of lava measuring up to a few meters in diameter, which follow ballistic trajectories before landing in the immediate vicinity up to a few kilometres from the vent. Although most people do not build homes in such proximity to a volcano, these often still partly molten rocks, which fall from the sky at temperatures of hundreds of degrees, pose a significant danger to nearby infrastructure and have caused injuries and fatalities among volcano enthusiasts and media personnel in the past. Additionally, they can ignite forest fires, thereby increasing the potential loss of life and property.
Pyroclastic Flow
Pyroclastic flows are hot mixtures of volcanic gases and sub-millimetre- to metre-sized lava fragments that travel down the flanks of a volcano like an avalanche, reaching distances of a few to hundreds of kilometres. They can be triggered by the collapse of an overloaded explosive eruption column or an over-steepened lava dome—a highly viscous "plug" of lava that forms at the vent of many dangerous volcanoes.
Pyroclastic flows occur suddenly and unpredictably and can reach temperatures of up to 1,000°C, traveling at speeds of up to 180 km/h. They are an unstoppable force and the most lethal volcanic danger, having caused more than 90,000 fatalities between 1600 and 2010, representing about one-third of all volcano-related fatalities [1].
The immense destructive forces of pyroclastic flows don´t only pose a direct threat to humans and livestock but also threaten infrastructures and buildings due to the high dynamic pressure and temperature. While hazard assessments can estimate the likely paths of the flows, which usually follow valleys around the volcano, many historic cases have shown that particularly strong flows can overflow onto topographic heights and reach areas previously deemed “relatively safe”.
Tragic events related to pyroclastic flows have occurred at volcanoes such as Merapi in Indonesia, Unzen in Japan, Vesuvius in Italy, and many others.

Lava Flow
Lava flows are formed by molten rock oozing directly from the vent or by the accumulation of many fluid lava bombs that are reunited in a flow. Because lava flows generally move slowly, people can walk away from them, making their fatality count low. There are a few volcanoes, however, whose rock chemistry is such that they produce lava flows that can move up to 20 km/h. The 2002 eruption at Nyiragongo volcano in the Democratic Republic of Congo is a rare example of a lava flow that caused casualties, resulting in approximately 100 deaths [4].
Lava flows generally cannot be stopped, and destroy everything in their path, either by igniting fires or by simply covering everything under a meter-thick layer of rock - although there have been some rare successes in diverting them. Most lava flows don't extend very far, stopping before they reach 10 kilometres from the vent. However, if the vent continues to feed them and they are not impeded by topography, the sea, or intrinsic cooling processes, lava flows have the potential to travel long distances.
The largest and most extensive lava flows are known as flood basalts. These colossal lava bodies can span up to 1,000 kilometres in length and width and can influence the climate due to the massive release of volcanic gases accompanying them [3]. The eruption that produced the immense lava flow field known as the "Deccan Traps" in India is believed to have played a role in the mass extinction event 65 million years ago, which resulted in the extinction of dinosaurs and numerous other species.
One of the most significant eruptions in recorded history occurred in 1783, when massive lava flows were emitted by the Laki fissure in Iceland. The volcanic gases associated with this eruption had a substantial impact on the global climate for several years. More recently, in 2018, the lava flows from Kilauea volcano in Hawaii caused an estimated US$536 million in damages. Another notable event took place in 2021 when the eruption of the Cumbre Vieja volcano on La Palma (an island off Spain) resulted in the destruction of farmland and over 1,600 buildings.

Landslide/Flank Collapse
Many volcanoes have steep flanks composed of loose materials, situated in tectonically dynamic environments, which makes them prone to flank collapses and major landslides. Landslides entail inherent hazards, but they can also have cascading effects. For example, if the volcano is located underwater or near a coastline, a flank collapse can generate a tsunami. As it removes overlying layers of rock, a landslide also has the potential to suddenly depressurise a magmatic reservoir, which can induce an eruption.
One of the most well-known examples of an eruption triggered by a landslide occurred in 1980 at Mount St Helens volcano in Washington State, USA. Prior to the eruption, magma was already rising beneath the volcano, exerting pressure on its flank. When the flank collapsed in a landslide, the pressure release caused the magma to erupt sideways from the volcano in a massive explosion [5]. This sideways eruption trajectory had never been observed before and tragically caught the staffed observatory unprepared. The Mount St Helens eruption is considered a landmark event in the field of volcanology, as it spurred extensive volcanological research and the development of the first mathematical models for eruptions.
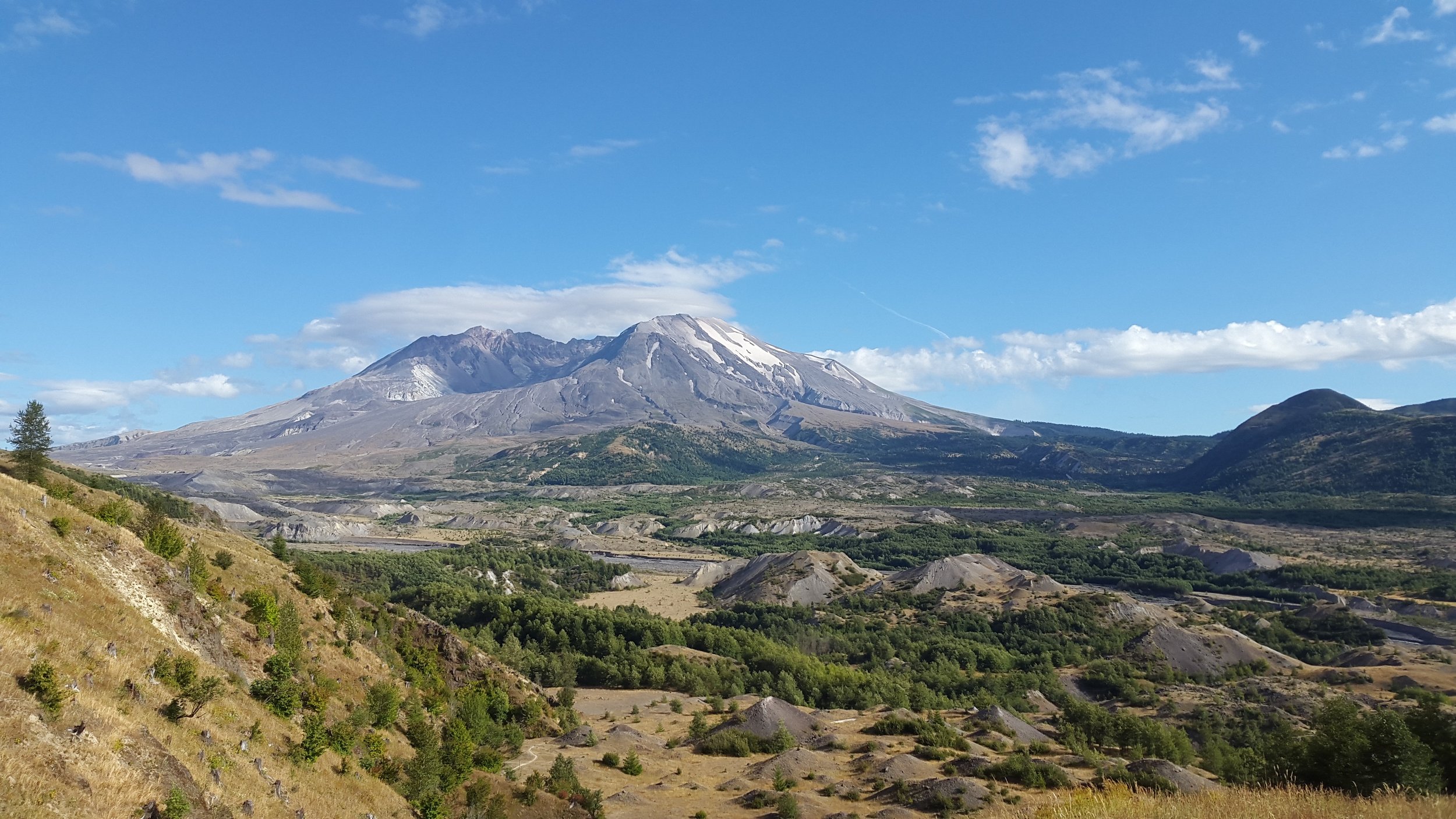
Lahar
The term "lahar" refers to volcanic mudflows that occur when intense rainfall interacts with a large amount of loose volcanic material, typically found in the steep terrain surrounding a volcano. While there is some correlation between lahar occurrence and rainfall, predicting their onset remains challenging, especially as they can happen independently of an ongoing eruption. Like pyroclastic flows, lahars are fast-moving and can cause extensive destruction, with the potential to travel hundreds of kilometres.
One of the most devastating lahar events took place at Nevado del Ruiz volcano in Colombia in 1985, claiming the lives of more than 23,000 people [1]. Another example is the town of Plymouth, the former capital of the island of Montserrat, which was destroyed by lahars and pyroclastic flows resulting from the eruption of Soufrière Hills volcano in the 1990s. The total damages caused by that eruption amounted to approximately US$500 million [2].
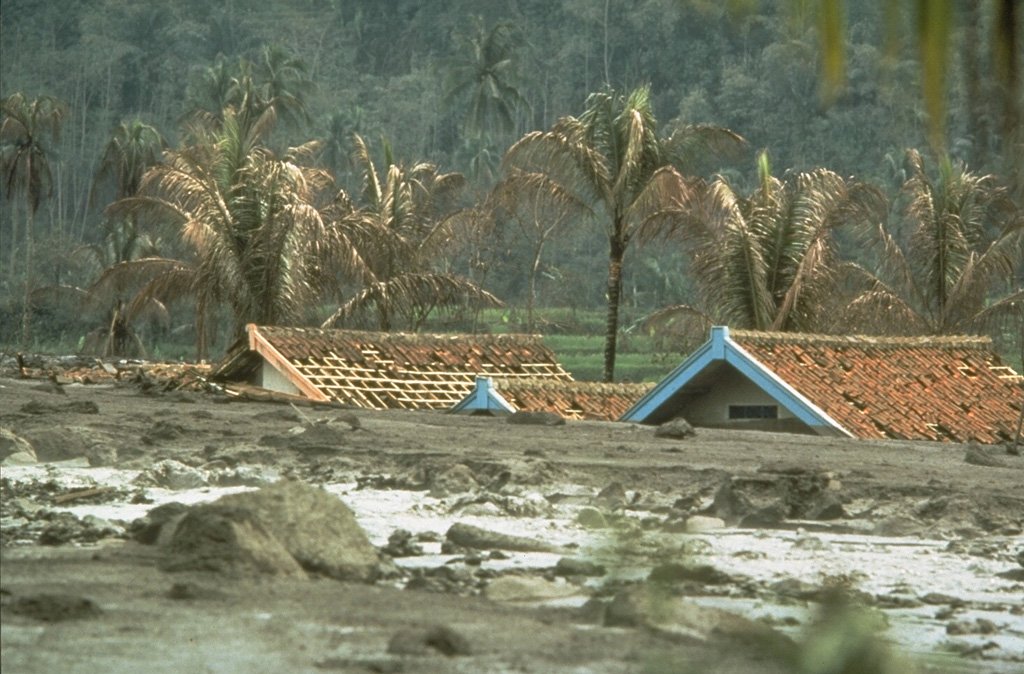
Volcanic Tsunami
An oceanic setting adds another dimension to the dangers associated with volcanic eruptions. Volcanic tsunamis, caused by submarine caldera collapses or the entry of pyroclastic flows or landslides into the sea, exemplify the cascading effects of volcanic activity. A staggering 20% of the fatalities caused by volcanoes between 1600 and 2010 can be attributed to volcanic tsunamis [1], which can traverse long distances and bring destruction from one side of the Pacific to the other.
Some of the most infamous and costly eruptions in history are renowned for their volcanic tsunamis, including the Krakatau eruption of 1883, which claimed 36,000 lives [1]. A more recent example is the eruption of the submarine volcano Hunga Tonga-Hunga Ha'apai in Tonga in January 2022. The combination of heavy ashfall and multiple tsunami waves that propagated across the Pacific caused severe damage, particularly in Tonga, where entire villages were destroyed, communication channels were disrupted for weeks, and numerous casualties occurred. The initial damage estimation by the World Bank for Tonga alone was approximately US$90 million composed of building and infrastructure damage, losses in the sectors of agriculture, forestry and fishery, and clean-up costs. The waves generated by this eruption also caused disruptions and damage in Japan, and tragically, even more than 10,000 km away, two deaths were attributed to this volcanic tsunami [7].
Volcanic Gases
Volcanoes not only act as pathways for lava but also release gases from deep within the Earth. While the gas plumes emitted from volcanic vents primarily consist of harmless water vapor, they also contain carbon dioxide (CO2), sulfur dioxide (SO2), and other halogenic gases. SO2 is responsible for the characteristic smell of rotten eggs experienced near a volcano when it reacts with water to form hydrogen sulfide (H2S). However, even in the absence of odour, SO2 poses a respiratory health risk to humans and can induce harmful acid rain, which adversely affects crops and livestock.
During larger eruptions, these gases can be injected high into the atmosphere, forming sulfate aerosols that have a significant impact on climate. This phenomenon explains why several major eruptions throughout Earth's history have led to global cooling, such as the 1815 Mt Tambora eruption in Indonesia, which caused the infamous "Year Without a Summer," and the 1783 Laki eruption mentioned earlier. Gases and aerosols emitted by volcanoes are dispersed by the wind, allowing them to travel great distances. In fact, sulfuric clouds from significant eruptions have been observed to encircle the entire globe.

Tephra Fallout
Explosive eruptions are driven by the rapid expansion of volcanic gases, which fragment the ascending lava into countless pieces and give rise to an eruptive column that can reach tens of kilometres in height. The mostly solidified, fragmented lava particles that are ejected during these highly energetic events and later deposited from the eruptive cloud are called "Tephra." Tephra is distinguished by size into bombs, lapilli, and the finest category, ash (smaller than 2 mm in diameter).
While bombs and larger lapilli have a comparatively limited range, ash and finer lapilli are widely dispersed by winds. An ash cloud can easily travel for hundreds and thousands of kilometres and even circle the globe during significant eruptions. Unlike volcanic flows that generally follow valleys, tephra is deposited like a blanket across the topography, leading to widespread damage. Even a mere 1 mm of ash fallout can significantly affect agriculture and critical infrastructure, such as transportation, energy, water, and telecommunication systems. When ash accumulation reaches 1 cm or more, it can damage buildings, and larger amounts can culminate in total roof collapses.
Airborne ash poses a significant respiratory health risk and threatens the aviation industry. The eruption of Eyjafjallajökull (Iceland) in 2010 serves as a famous example, as it caused a total loss of USD 2 billion solely in the aviation sector due to delays, rerouting, and cancellations caused by the ash cloud [6]. Another notable eruption, the 1991 Pinatubo eruption in the Philippines, resulted in a total loss of USD 750 million due to tephra fallout and pyroclastic flows [8]. Many cities worldwide are at risk of volcanic ash fallout, and according to Swiss Re, a volcanic eruption could cost large cities up to USD 30 billion in economic losses [9].
Overall, tephra fallout from explosive volcanic eruptions poses significant threats to various aspects of human life, including infrastructure, agriculture, public health, and economic stability. This highlights the importance of monitoring and preparedness measures to mitigate the potential impact of tephra deposition.

Despite their majestic beauty, volcanoes pose serious threats. Most of the losses they have incurred throughout history were uninsured. Despite the significant and potentially long-lasting negative economic impact of volcanic eruptions, available products on the insurance market are rare or non-existent, and the insurance gap for recent eruptions has been between 50 and 100% [10]. According to Munich Re, about half of the global volcanic losses of 12 bn US$ between 1980 and 2019 were inflicted on Asian countries. The Asia-Pacific region is one of the most volcanically active on the planet and will be the focus of our next "Geographic Spotlight” in the Volcano catbond article series.
References:
- Auker, M. et al. (2013) A statistical analysis of the global historical volcanic fatalities record. Journal of Applied Volcanology, 2:2. https://doi.org/10.1186/2191-5040-2-2
- https://uwiseismic.com/volcanoes/caribbean-volcanoes/
- Newhall, C.G. (2015) Volcanology 101. In G. Schubert (Ed.), Treatise on Geophysics (Second Edition) (pp. 355-387). Elsevier. https://doi.org/10.1016/B978-0-444-53802-4.00080-4
- Favalli, M. et al. (2009) Lava flow hazard at Nyiragongo volcano, D.R.C. Bull Volcanol, 71:363-374. https://doi.org/0.1007/s00445-008-0233-y
- https://www.usgs.gov/volcanoes/mount-st.-helens/1980-cataclysmic-eruption
- Jenkins, S.F. et al. (2015) Volcanic ash fall hazard and risk: Technical Background Paper for the UNISDR 2015 Global Assessment Report on Disaster Risk Reduction. Global Volcano Model and IAVCEI. www.preventionweb.net/english/hyogo/gar. DOI: 10.1017/CBO9781316276273.005
- The Global Volcanism Program https://volcano.si.edu/
- Annen and Wagner (2003) The Impact of Volcanic Eruptions During the 1990s. Nat Hazards Rev, 4:169-175
- https://www.swissre.com/dam/jcr:02550b19-f9a2-45f2-9288-377d25952b5b/Swiss+Re_Volcano_PR_en.pdf
- Smolka, A., and Käser, M. (2015) Chapter 12 - Volcanic Risks and Insurance. In Shroder, J.F., and Papale, P. (Eds.), Volcanic Hazards, Risks and Disasters (pp. 301-314), in Hazards and Disasters Series, Elsevier. DOI: 10.1016/B978-0-12-396453-3.00012-5